What is a Stem Cell?
What is a Stem Cell? A Stem cell is an undifferentiated cell which differentiates to become any type of cell and from there further sub-divides. Scientists have recently utilized stem cells in regenerative and rejuvenative medicine. Stem cells transplanted into damaged human bodies will eventually contribute to a “De-Hospitalized Society”. The society has less drugs and less hospitals than now.
Stem cell Types:
- ES Cell (embryonic stem cell)
- iPS Cell (induced pluripotent stem cell)
- Adult Stem Cell
Let’s survey historical aspects of stem cell.
I. Epigenetic Landscape
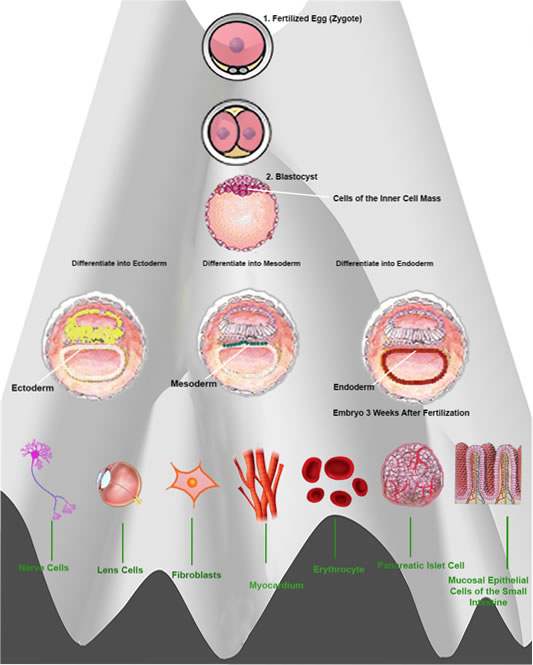
Cells develop from only one fertilized egg — zygote. A “Totipotent Cell” with the ability to differentiate into more than 220 cell types. except placenta. But its Totipotency, (which represents the cell with the greatest differentiation potential) is not retained during all the various stages of cell sub-division.
The first sub-division provides 2 Totipotent cells. The second 4 cells, retain Totipotency But the following 8 cells no longer retain the ability to differentiate into all types of cells. Further sub-divisions differentiate into specialized cells such as fibroblasts, erythrocytes, nerve cells, intestinal mucosal epithelial cells and pancreatic islet cells, etc. but following 6 to 7 full further cell sub-divisions (about 5 days after fertilization), the embryo becomes a “Blastocyst” and possesses “Inner Cell Mass (ICM) “
ICM cells can differentiate into any cell type, except placenta — pluripotency. 3 weeks after fertilization, the cells of the inner cell mass differentiate into ectoderm, mesoderm and endoderm. In the 1950s, this differentiation was thought to be irreversible, being described as a ball rolling down a hill and technically known as ” Epigenetic Landscape”.
This was until 1962 when John Gurdon created clone frogs and then in 1996 Ian Wilmut created “Dolly ” the cloned sheep. Scientists had previously thought stem cells could not have pluripotency once dispersed into a specialized cell. however, in nature, even once differentiated, some cells can again differentiate and this phenomenon is known to science.
II. Cells of Planaria
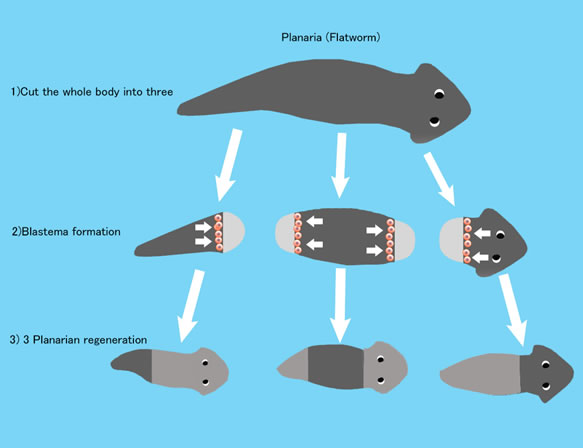
The stem cells of humans have limited ability to differentiate. But for example, Planaria that is a small flatworm living in rivers has amazing ability to differentiate into any organs, even into itself. When it is chopped into 3 fragments like picture, each fragment regenerates to a perfect Planaria. There is a record that 1/279 fragment of one Planaria regenerated to perfect Planarias. When Planaria grows to a certain size, it divides itself to two fragments. And each fragment grows to a perfect Planaria. For Planaria, division and regeneration are mechanism for reproduction. But under some circumstances Planaria chooses sexual reproduction.
III. Cells of Plant
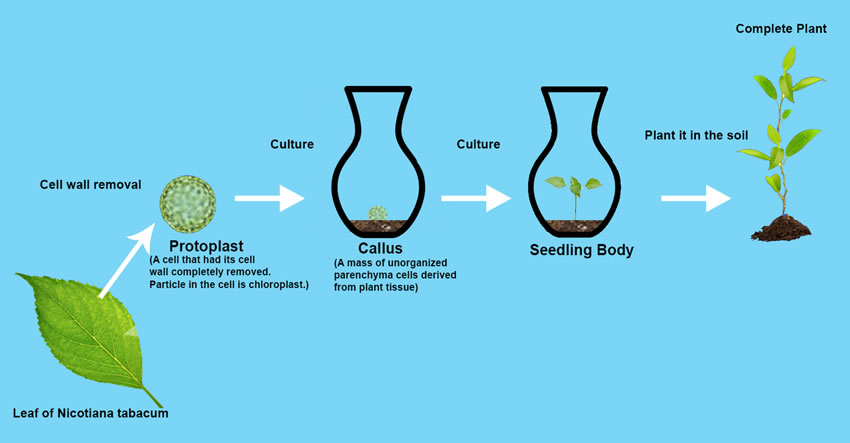
A Plant can regenerate itself from one already differentiated cell. An Enzyme mixture of Cellulase Onozuka R-10 and Macerozyme R-10 dissolves tobacco plant (Nicotiana tabacum) leaf cell walls, and cells become leaf protoplasts (= cell without cell walls). When protoplasts are cultivated under appropriate condition, the protoplasts grow to a seedling plant through plant callus (= mass of unorganized parenchyma cells). When the seedling plant is planted in soil, it grows to a perfect tobacco plant. Two British scientists proved biological technique could change Epigenetic Landscape.
IV. Frog of John Gurdon
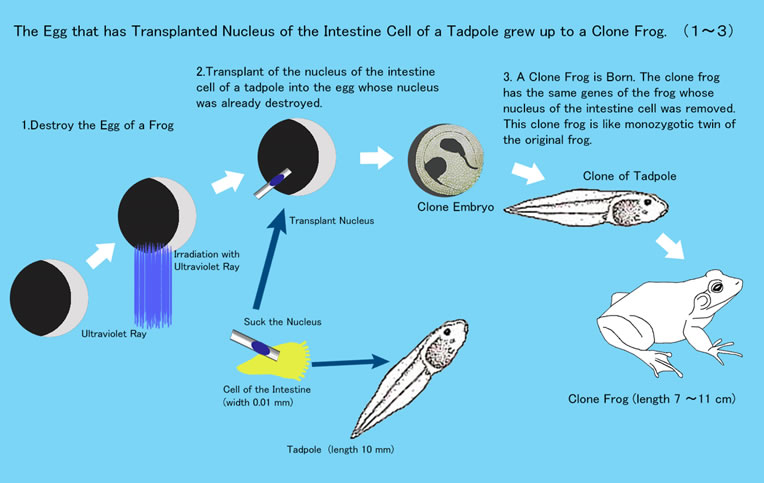
Gurdon created cloned frogs.
- He destroyed the nucleus of Frog A ovum by ultraviolet rays.
- He removed the intestinal cell “tadpole nucleus” of Frog B which was transplanted into Frog A’s ovum, the nucleus of which was already destroyed by ultraviolet rays. This new ovum became Clone Embryo B which grew into Frog B’s tadpole was later to become cloned adult Frog B.
V. Ian Wilmut's "Dolly" The Cloned Sheep
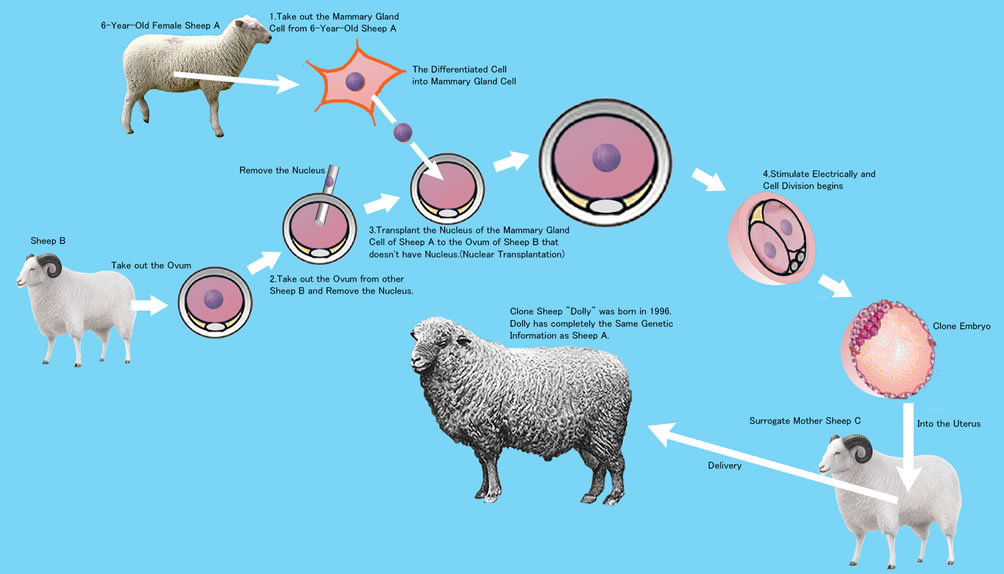
Wilmut used a cell nucleus which had been differentiated in the mammary gland.
- He took out the ovum from Sheep A, and removed its nucleus.
- From Sheep B he removed the mammary gland cell nucleus.
- Into the ovum of Sheep A, he transplanted the nucleus of Sheep B.
- The Sheep A ovum was then electrically stimulated.
- The stimulated cell divided to became the cloned embryo of Sheep B.
- The cloned Sheep B embryo was transplanted into the uterus of Sheep C.
- Sheep C gave birth to Cloned Sheep B. This sheep was named “Dolly”.
VI. ES Cells (embryonic stem cells)
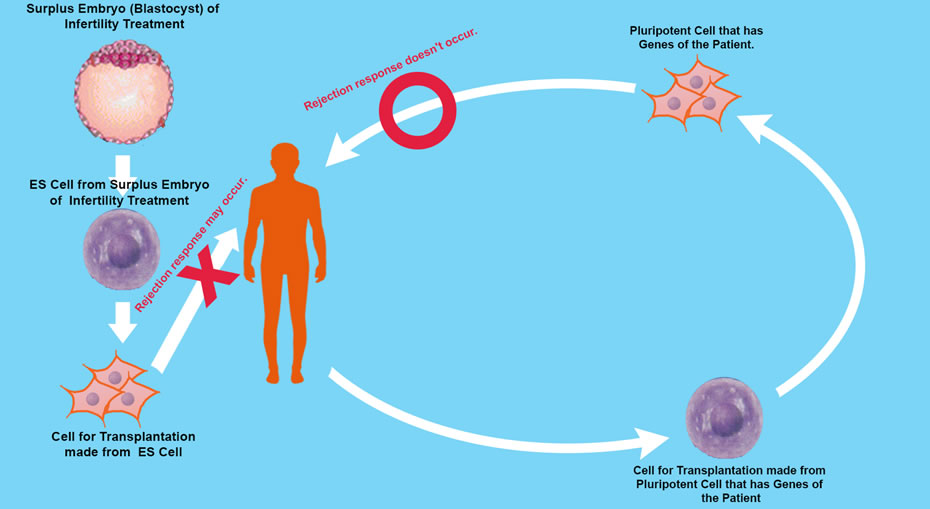
Human embryos reach “Blastocyst” about 5 days’ post fertilization. The Blastocyst possesses an “Inner Cell Mass (ICM) ” In 1981 Martin Evans succeeded in culturing mouse ICM cells. These cells are capable of propagating themselves indefinitely in an undifferentiated state, and they can differentiate into any type of cell except placenta. These cells are pluripotent, and known as Embryonic Stem Cell (ES Cells).
James Thomson created ES Cells from Monkey embryo in 1995 and later in 1998 created ES Cells from human embryo.
However, research into ES Cell needs further study due to an ethical dilemma, that in order to isolate inner cells from the blastocyst, the blastocyst is destroyed, so is the embryo at pre-implantation stage to be considered human? and even if not, do we have the right to destroy human potential growth.
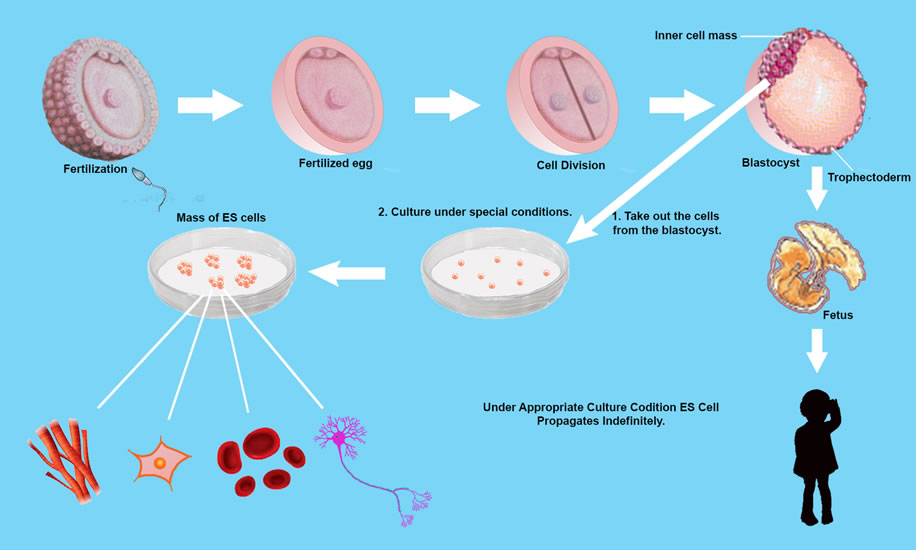
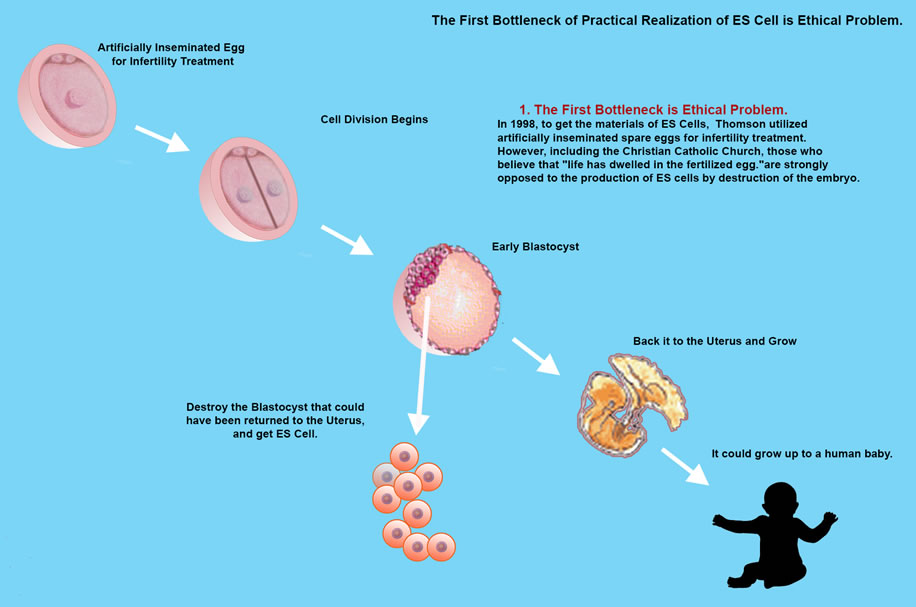
VII. iPS Cells (Shinya Yamanaka)
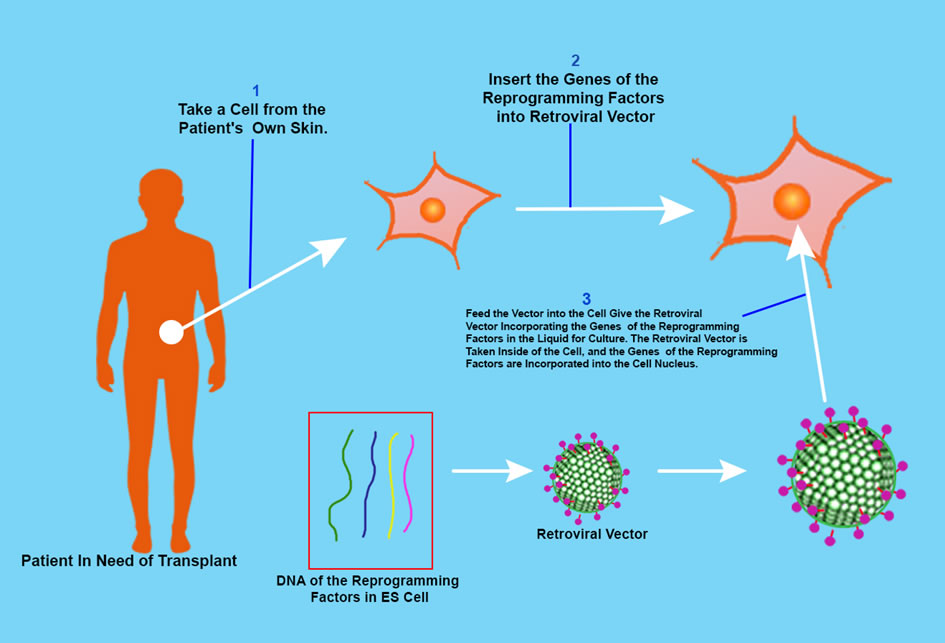
The ethical issue of ES Cells can be by-passed by using induced pluripotent stem cells. The iPS cell is a pluripotent stem cell which can be generated directly from adult cells, not from human embryos, and in 2006, Shinya Yamanaka and his team in Kyoto University created iPS cells from mouse fibroblasts.
He hypothesized that the genes playing a pivotal role in the function of ES Cells could induce an embryonic state in adult cells. But how many are the genes? The human has 20 – 25 thousand genes. Yamanaka researched and found 24 genes which were important for the characteristic protein of human ES Cells, and then used retroviruses to deliver all 24 genes into mouse fibroblasts, and the fibroblasts were able to propagate indefinitely. These iPS Cells were pluripotent like ES Cells.
Yamanaka removed one factor at a time from the 24 factors to identify the necessary genes for reprogramming and by this process he identified 4 factors — Oct3/4, Sox2m, Klf4 and c-Myc – named the “Yamanaka Factors”, and Later he found c-Myc was not needed for reprogramming, but without c-Myc the process took longer and was inefficient. He was awarded the Nobel Prize for Physiology or Medicine in 2012 with John Gurdon (above mentioned) for the discovery that mature cells can be converted to stem cells. And in 2013 he was awarded the $3 million Breakthrough Prize in Life Sciences for his work.
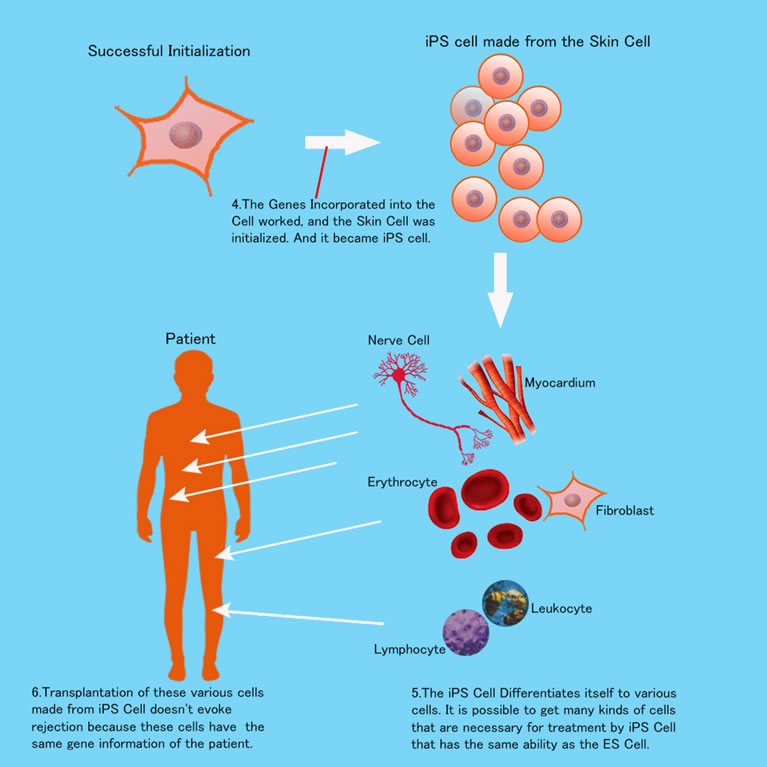
A strong concern of the iPS researchers was if iPS Cell differentiation caused cancerous cells. But this issue has almost been resolved completely through rigorous study. And many clinical human applications are now carried out in Japan. For example, in 2014 retina transplantation by iPS Cells was successfully carried out for age-related macular degeneration. And cells did not differentiate into cancer cells. In my next page, I write more about these applications.
iPS Cells are useful not just for regenerative medicine but for drug discoveries or development. Because it is very easy for researchers to recreate special cells which cause special diseases, in a petri dish — Alzheimer’s disease, Parkinson’s disease, ALS (Amyotrophic Lateral Sclerosis), Schizophrenia, etc.
For example, “Achondroplasia” which is caused by mutation in fibroblast growth factor receptor 3. This is a common cause of dwarfism. Researchers made iPS Cells from skin fibroblasts of 3 patients with achondroplasia then allowed the iPS Cells to differentiate into chondrocytes over 2 to 3 weeks.
Chondrocytes in the petri dish secreted about themselves an extracellular matrix which is characteristic of chondrocytes and made a mass.
Compared to the chondrocytes of healthy people, these patient’s chondrocytes grew slowly, and the researchers tried thousands of drugs one by one to cure the abnormal chondrocytes from the petri dish specimens. Then finally, and with much surprise, they found the “Statin drug” was effective and able to cure the abnormal chondrocytes of achondroplasia patients. Why surprise? Because Statin drugs are for lowering cholesterol, and nobody expected cholesterol lowering drugs to be effective against achondroplasia.
In the next pages I explain in more detail about practical and clinical uses of iPS Cells.
VIII. Adult Stem Cell (Somatic Stem Cells or Tissue Stem Cell)
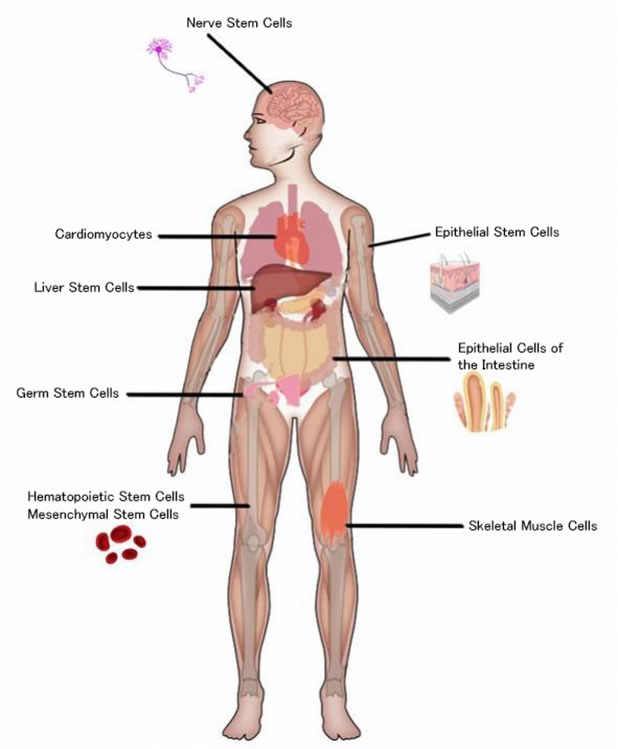
Adult Stem Cells are undifferentiated cells found throughout the body such as in bone marrow, and umbilical cord blood, and the mammary gland, and the surface of the small and large intestines, the adipose tissue, the lining of the nose, the testicles, and the hair follicle, between the basement membrane and the sarcolemma of muscle fibers (Satellite Cells), etc.
These cells are multipotent cells that have less ability to differentiate into specialized cells than pluripotent cells. The adult stem cell from the bone marrow, called Hematopoietic Stem Cell (HSC), was discovered in the 1960s by two Canadian biologists, James Till and Ernest McCulloch, and has been used clinically to cure various blood diseases, such as leukemia, malignant lymphoma, multiple myeloma, etc. Clinically a very important cell. But for regenerative medicine, it needs much practical work to obtain stem cells from bone marrow, and requires general anesthesia, however scientists recently have found it easier to obtain these cells.
This is by ASC (adipose-derived stem cell) from our fat. The first scientific reports on ASC were made by an American scientist, Patricia Zuk (UCLA), in 2001. She reported the presence of mesenchymal stem cells in the fat tissues, and as they have a faster growth rate, these cells are expected to be advantageous for regenerative medicine.
ASC can differentiate into muscle, bone, cartilage, liver, adipose cell (lipid cell). And besides the advantages as stem cell, ASC secrets exsosome (nano size particles) that contain enzymes which dissolve beta-amyloid of Alzheimer’s disease. The efficacy is 8 times more potent than the enzymes secreted by the exssome of the bone marrow.
ASC is now aggressively researched in Japan for practical uses. It will be used to treat many diseases such as Alzheimer’s, Parkinson’s, and diseases of the liver and kidneys, and periodontal disease, and more.
In Japan Tottori University Medical School researchers have established the technique of breast reconstruction by ASC after mastectomy due to cancer. They operate and inject ASC into the patients depressed breast. The breast recovers to the original shape within three months. This is not silicon, but the patient’s own cells. Quite natural. No rejection. The cost of this treatment will be covered by health insurance within three years in Japan.
And Doctors at the Nagoya University Medical School use ASC against urinary incontinence stress. The sphincter function of the urethra often weakens due to aging, delivery, and some bladder diseases. ASC is injected around the patient’s urethra to strengthen the smooth muscle.
However, some side effects may occur by use of ASC. For example, male prostate hyperplasia and female endometriosis. Issues not studied in depth. So for now until the side effects have been dealt with, we should wait for general anti-aging treatments.
IX. Direct Reprogramming. "A Newer Approach"
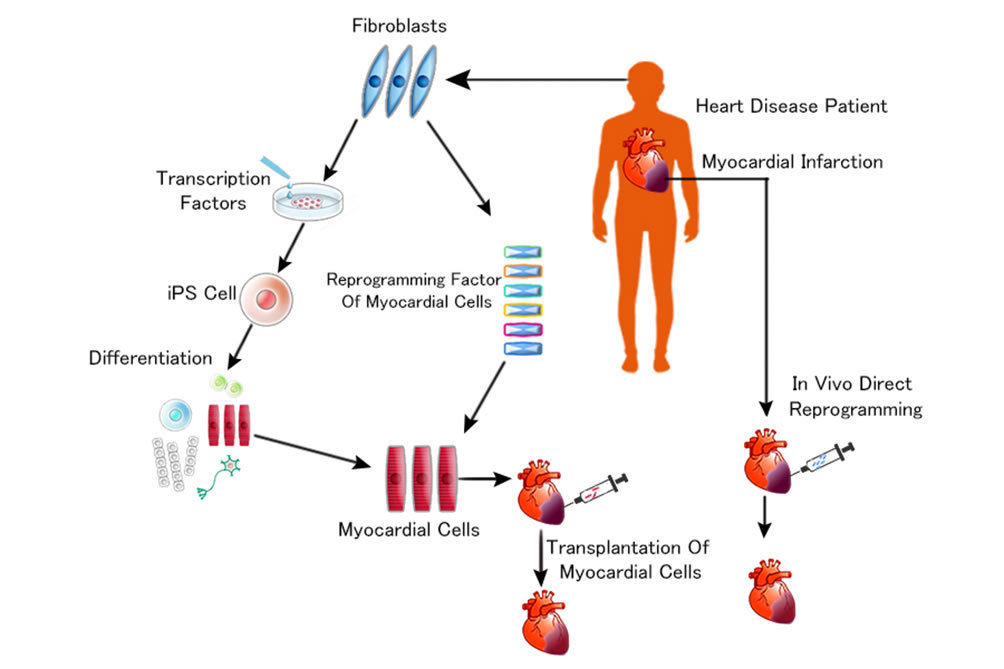
Recently it was found possible to induce, directly from somatic cells, not only iPS but nerve cells, hepatocyte cells, myocardium cells, cartilage cells, and many varied cells by introducing the specific key transcription factors in cell differentiation, which means that through by-passing of pluripotent stem cells it is possible to induce differentiated specific cells from somatic cells. This is called “Direct Reprogramming”, and the most exciting research for example is: myocardial reprogramming in vivo, where Doctors inject patient’s fibroblasts with transcription factors to the infarcted lesion of the heart.
There the fibroblasts differentiate into myocardium (heart muscle). So, simple and quick. And this regenerative medical technique is under development mainly in Japan and the United States and as this technique is established, lots of heart surgeries will become obsolete and this is also true of brain surgery. The numbers of hospitals will eventually reduce and cost of time consuming surgeries will lower and this will lead on to a “De-Hospitalized Society”.
X. In Conclusion
For a basic understanding of stem cell mechanisms: ES Cell is studied along with iPS Cell and ASC. But ES Cell has an ethical dilemma and this issue shall not be overcome by science. So, it cannot be used for the treatment of human diseases. Direct Reprogramming: Wonderful technology. But it may take 10 or 20 years more to accomplish it. Therefore, at this moment, iPS Cell and ASC are most realistic medical tools for those who are suffering from degenerative diseases and wish rejuvenation.
Practical Applications of iPS Cells
Stem cells and induced pluripotent stem cells (iPS or iPSCs) have found their usage in a large number of human welfare such as drug development, toxicity tests, organ/ tissue regeneration and repair etc. Induced pluripotent stem cells (iPSCs) could also be of great use in studying molecular mechanism of many diseases. Many diseases have been modeled by using iPSCs for better understanding of their etiology which maybe further utilized for developing putative treatments for these diseases. The use of iPSCs may eliminate the chances of immune rejection as patient specific cells may be used for transplantation in various engraftment processes. Moreover, iPS technology has been employed in various diseases for disease modeling and gene therapy. The technique offers benefits over other similar techniques such as animal models. Many toxic compounds (different chemical compounds, pharmaceutical drugs, other hazardous chemicals, or environmental conditions) which are encountered by humans and newly designed drugs may be evaluated for toxicity and effects by using iPSCs. The applications of stem cells and especially iPSCs in regenerative medicine, disease modeling, and drug discovery are enormous and should be explored in a more comprehensive manner.
In this web presentation you read 6 major applications of Stem cells and IPS cells:
- Uses in Regenerative medicine (tissue/organ regeneration)
- Uses in Disease modelling
- Uses in Drug Discovery / Development
- Uses in Drug testing/ Toxicity Testing
- Uses in Tissue repair
- Uses in Gene Therapy
Advantages of iPS technology over other conventional therapies:
- The ethical issues are avoided by the use of iPSCs
iPSCs have solved the controversy over the destruction of embryos associated with the use of ES Cells (embryonic stem cells). - Reduced chances of immuno-rejection
iPSCs are generated from the somatic cells of one’s own body and hence there is no risk of immuno-rejection of these autologous cells. - Throughput screening for predicting toxicity/therapeutic responses of newly developed drugs
The concept of using iPSCs to predict toxicology and therapeutic responses of drugs in based on the property of iPSCs to continuously self- renew which make it possible to generate libraries and their ability to give rise to all types of body cells. - Lowering the overall cost and risk of clinical trials
The cost used for the clinical trials could be reduced by using iPSCs to provide the toxicity details of the drug by different cytotoxicity assays. The use of iPSCs for these tests may cut the cost associated with providing animal models which will ultimately decrease the overall costs of the clinical trials. - Development of a personalized approach for administration of drugs
As, iPSCs are derived from individual patients, these offer scientists an opportunity for modeling diseases on a patient-by-patient basis. This enables screening the genomic differences between individuals that may help in the progression of disease, and the screening of pharmacological agents to find the ideal one for each individual. - Gene targeting and correction technologies (gene therapy)
Reprogramming of somatic cells from any genetic background to iPSCs has allowed the generation of cell lines possessing disease-causing mutations.
Uses in Regenerative Medicine
The application of iPSCs for generating tissues and organ grafts can solve many problems in organ transplants and improve the quality of life for millions of patients. iPSCs can be directed to generate specific cell types and can be used to replace ailing or degenerating tissues. The greatest advantage of this technique is that it overcomes graft-to-host incompatibility. It is for this reason that graft rejection by the hosts immune system decrease significantly. There are about 90,000 patients in the US transplant-waiting list, who need medical assistance, which can be addressed by stem cell technologies.
Kidney Regeneration
iPSCs technology can be used to transform terminally differentiated skin cells into kidney organoids which are functionally and structurally similar to those of kidney tissue in vivo. During kidneys healing process, a progenitor stem cell needs to become 20 types of cells, required for waste excretion, pH regulation, and restoration of water and electrolytic ions. The ex vivo kidney organoids are similar to fetal first-trimester kidneys for their structure and physiology. They can also be served as model for nephrotoxicity screening of drugs, disease modelling, and organ transplantation. However, there are many hurdles coming to the point of generation of fully functional kidney that can be dreamed for the near future.
Liver Regeneration
iPSC-Liver buds have been developed from a mixture of three different kinds of stem cells, i.e., hepatocytes (for liver function) coaxed from iPSCs; endothelial stem cells (to form lining of blood vessels) from umbilical cord blood; and mesenchymal stem cells (to form connective tissue). This approach is like mimicking the fetal development and formation of a complex organ. In one experiment, after growing in vitro for a few days, the liver buds were transplanted into mice where the ‘liver’ quickly connected with the host blood vessels and continued to grow. It also performed regular liver functions including metabolizing drugs and producing liver-specific proteins. Further studies will monitor the longevity of the transplanted organ in the host body (ability to integrate or avoid rejection) and whether it will transform into tumors. Using this method, cells from one mouse could be used to test 1,000 drug compounds to treat liver disease, and reduce animal use by up to 50,000.
Visual acuity Regeneration
Loss of neurons in age-related macular degeneration (ARMD) is the common cause of blindness. At preclinical level, transplantation of iPSCs derived neuronal progenitor cells (NPCs) in rat limits progression of disease through generation of 5-6 layers of photoreceptor nuclei, restoring visual acuity.
Y. Tsai, B. Lu, B. Bakondi et al., “Human iPSC-derived neural progenitors preserve vision in an AMD-like model,” STEM CELLS, vol. 33, no. 8, pp. 2537–2549, 2015.
Neurons Regeneration
The high order brain functions, like emotions, anxiety, sleep, depression, appetite, breathing heartbeats, and so forth, are regulated by serotonin neurons. Generation of serotonin neurons occurs prior to birth, which are post mitotic in their nature. Any sort of developmental defect and degeneration of serotonin neurons might lead to neuronal disorders like bipolar disorder, depression, and schizophrenia-like psychiatric conditions. Manipulation of signaling in human iPSCs in defined culture conditions leads to an in vitro differentiation of iPSCs to serotonin-like neurons. These iPSCs-neurons primarily localize to rhombomere 2-3 segment of rostral raphe nucleus, exhibit electrophysiological properties similar to serotonin neurons, express hydroxylase 2, the developmental marker, and release serotonin in dose and time dependent manner. Transplantation of these neurons might cure from schizophrenia, bipolar disorder, and other neuropathological conditions.
J. Lu, X. Zhong, H. Liu et al., “Generation of serotonin neurons from human pluripotent stem cells,” Nature Biotechnology, vol. 34, no. 1, pp. 89–94, 2016.
Placenta Regeneration
Placenta, the cordial connection between mother and developing fetus, gets degenerated in certain pathophysiological conditions. Nuclear programming of OCT4 knock-out (KO) and wild type (WT) mice fibroblast through transient expression of GATA3, EOMES, TFAP2C, and +/− cMYC generates transgene independent trophoblast stem-like cells (iTSCs), which are highly similar to blastocyst derived TSCs for DNA methylation, H3K7ac, nucleosome deposition of H2A.X, and other epigenetic markings. Chimeric differentiation of iTSCs specifically gives rise to hemorrhagic lineages and placental tissue, bypassing pluripotency phase, opening an avenue for generation of fully functional placenta for human.
H. Benchetrit, S. Herman, N. Van Wietmarschen et al., “Extensive nuclear reprogramming underlies lineage conversion into functional trophoblast stem-like cells,” Cell Stem Cell, vol. 17, no. 5, pp. 543–556, 2015.
Lungs Regeneration
Degeneration of other organs and tissues also has been reported, like degeneration of lungs which might occur due to tuberculosis infection, fibrosis, and cancer. The underlying etiology for lung degeneration can be explained through organoid culture. Coaxing of iPSC into inert biomaterial and defined culture leads to formation of lung organoids that consisted of epithelial and mesenchymal cells, which can survive in culture for months. These organoids are miniature lung, resemble tissues of large airways and alveoli, and can be used for lung developmental studies and screening of antituberculotic and anticancer drugs.
B. R. Dye, D. R. Hill, M. A. Ferguson et al., “In vitro generation of human pluripotent stem cell derived lung organoids,” eLife, vol. 4, Article ID e05098, pp. 1–25, 2015.
Chronic Wound Healing
Chronic wounds are rarely seen in otherwise healthy individuals; they are often associated with diabetes, obesity or old age. It has been estimated that 1-2% of people in developed countries suffer from chronic wounds in their lifetime [i].Current methods of wound management are palliative, but their ineffectiveness for complex wounds is an ongoing clinical problem. Healthcare systems are in desperate need of alternative therapies. Stem cells/ iPSCs are known to tremendously influence normal cell and tissue repair/regeneration, which is why a large proportion of research focuses on stem cells as the answer to treating chronic wounds. Self-renewing characteristics and multipotent differentiation potential of stem cells make them ideal candidates for the treatment of chronic wounds. Stem cell-based therapies bring about their effectiveness via a number of mechanisms. Stem cells can differentiate into new cells, secrete trophic factors, promote angiogenesis, modulate the immune system, improve wound closure, and also help in the development of new extracellular matrix (ECM).
- F. Gottrup. A specialized wound-healing center concept: the importance of a multidisciplinary department structure and surgical treatment facilities in the treatment of chronic wounds. The American Journal of Surgery, vol. 187, no. 5, pp. S38– S43,2004.
- http://www.fiercebiotech.com/r-d/researchers-repair-retinas-mice-virus-free-stem-cells
Disease Modelling
The use of stem cells especially iPSCs for disease modeling is based on the fact that these cells are capable of self-renewing and that these cells can differentiate into all types of cells of the human body which can be utilized for the preparation of different disease models to study those diseases. Moreover, a patient specific iPSC could be of enormous use as far as development of specific therapeutics regimen/drug is concerned. By combining 3D culture with extracellular matrix proteins, in-vivo microenvironment can be mimicked. iPSCs have helped out in studying various mechanisms that play role in different diseases, a few have been described below.
The following findings from modeling different diseases help out in knowing the molecular mechanisms underlying the disease better, which ultimately carries the work forward to knowing the disease better for the development of a treatment.
Neurogenerative diseases
Parkinson’s Disease (PD) is a very common neurodegenerative disease, in which, dopaminergic neurons of substantia nigra (a structure in mid brain) get lost and formation of Lewy’s bodies (inclusions in the cytoplasm of neurons all over the body) occurs. Treatment of this disease had not been possible due to the reason that by the time, Parkinson Disease gets clinically manifested, the neurons have already lost, which makes it very difficult to study the underlying mechanisms of Parkinson disease so as to develop a treatment of it. In such a situation, iPSCs can be used and experiments have also been carried out in this aspect. Devine et al. developed iPSCs from fibroblasts taken from a Parkinson disease affected person possessing triplication of Synuclein gene by the transduction of four basic transcription factors. These iPSCs were then directed to differentiate into dopaminergic neurons in vitro for the study of Parkinson Disease.Another research by Professor Atsushi Takahashi of Kyoto University iPS cell research institute transplanted nerve cells made from human iPS cells to Parkinson’s disease monkey on August 30, 2017 claimed to alleviate the Parkinson disease symptom (hand tremor). The research results were published in English Science Journal, Nature (electronic version). The research team created nerve cells that emitted dopamine from iPS cells based of Parkinson’s disease patients. This was transplanted into the brain of cynomolgus monkeys reproducing the symptoms of Parkinson’s disease. Observing the one year course after transplantation, the symptoms of Parkinson ‘s disease such as trembling and reduced exercise ability were alleviated over time.
Devine, Michael J., et al. “Parkinson’s disease induced pluripotent stem cells with triplication of the α-synuclein locus.” Nature communications 2 (2011): 440
Alzheimer’s Disease
Recent preclinical evidence suggests that stem cells can be used to treat or model Alzheimer’s Disease. The mechanisms of stem cell based therapies for Alzheimer’s Disease include stem cell mediated neuroprotection and trophic actions, anti-amyloidogenesis, beneficial immune modulation, and the replacement of the lost neurons. iPSCs have been recently used to model investigate sporadic and familial Alzheimer’s Disease pathogenesis, and screen for anti- Alzheimer’s Disease, drugs.
Fan, Xiaotang, et al. “Stem‐Cell Challenges in the Treatment of Alzheimer’s Disease: A Long Way from Bench to Bedside.” Medicinal research reviews 34.5 (2014): 957-978.
Down’s syndrome
Briggs et al. used iPSCs for the identification of molecular networks that drive the different aspects related to pathogenesis in Down’s Syndrome. iPSCs in combination with microarray and RNA sequencing technology, can be used to generate phenotype-genotype maps of complex diseases by linking various defects with phenotypes, like in Down’s Syndrome using Chromosome engineering of Down Syndrome-iPSCs
Briggs, James A., et al. “Integration‐free induced pluripotent stem cells model genetic and neural developmental features of down syndrome etiology.” Stem Cells 31.3 (2013): 467-478.
Study of Prostate and Urinary Tract diseases
Moad et al. used human prostate and urinary tract cells for the formation of iPSCs and further for studying the mechanisms that regulate the differentiation of prostate and urinary tract cells. With their study, they reported the first successful reprogramming of bladder, prostate and ureter stromal fibroblasts into a pluripotent state and concluded that iPSCs generated from prostate and urinary tract had better efficiency of differentiation to cells of prostate and urinary tract as compared to iPSCs derived from skin fibroblasts which showed that organ of origin plays an important role in terms of efficiency of differentiation.
Moad, Mohammad, et al. “A novel model of urinary tract differentiation, tissue regeneration, and disease: reprogramming human prostate and bladder cells into induced pluripotent stem cells.” European urology 64.5 (2013): 753-761.
Other deficiency diseases – Various types of diseases which are caused by some deficiency have been studied by using iPSCs. Park et al. used iPSCs from patients of various diseases like Adenosine Deaminase Deficiency-related Severe Combined ImmunoDeficiency (ADA-SCID), Shwachman-Bodian-Diamond syndrome (SBDS), Gaucher disease (GD) type III for the study of disease models and drug discovery.
List of diseases where iPSCs have been used for gene therapy and disease modeling.
Disease/syndrome | Cause(s) | Features | Cells used for iPSC generation |
---|---|---|---|
Becker type muscular dystrophy (BMD) | Mutation in dystrophin gene | Loss of walking ability, but progression slower than DMD | Fibroblasts |
Downs syndrome/trisomy 21 | Trisomy of chromosome 21 | Cardiac and cognitive defects, premature Alzheimers disease and aging, dysmorphic facial features | Fibroblasts |
Familial dysautonomia (FD) or Riley-Day syndrome | Autosomal recessive disorder caused by a single mutation in exon 20 in I-K-B kinase complex associated protein (IKBKAP) gene | Dysfunction of small fiber sensory neurons | Fibroblasts |
Childhood cerebral adreno leuko dystrophy (CCALD) | Mutation in ABCD1 gene | Adrenal cortex, nervous system and testes get affected, leading to rapid cerebral demyelination and adrenocortical atrophy. | Skin fibroblasts |
Rett’s syndrome | Classic form caused by loss-of-function mutation in Methyl-CpG-binding protein 2 (MECP2) gene on the X – chromosome, variants caused by mutations in FOXG1 or CDKL1 on chromosome 14 and X-chromosome, respectively | Neurocognitive regression and autistic behavior | Fibroblasts |
Duchenne type muscular dystrophy (DMD) | Biochemical and genetic defects in Dystrophin-glycoprotein complex | Loss of walking ability | Tail tip fibroblasts (mouse) |
Alzheimer disease | Duplication of amyloid β precursor protein (APP) | Presence of neurofibrillary tangles and amyloid plaques in the brain | Fibroblasts |
LEOPARD syndrome | Mutation in protein tyrosine phosphatase non-receptor type 11 (PTPN11) gene | Increased failure of bone marrow, pulmonary fibrosis and cancer, oral leykoplakia, abnormal skin pigmentation and nail dystrophy | Fibroblasts |
α1-antitrypsin deficiency | Mutation in α1-antitrypsin (A1AT) gene | misfolded α1-antitrypsin gets aggregated in the endoplasmic reticulum | Dermal fibroblasts |
Parkinson’s Disease (PD) | Familial forms caused by α-synuclein, ubiquitin carboxy terminal hydroxylase L1, parkin, DJ-1, putative serine threonine kinase 1 and leucine rich repeat kinase 2 | Loss in nigrostriated dopaminergic neurons in substantia nigra; presence of Lewy bodies | Dermal fibroblasts of patient with idiopathic PD |
Huntington disease (HD) | CAG repeats (36 or more) in the first exon of htt gene gets expanded abnormally | Degeneration in striatum and cerebral cortex | Fibroblasts |
ALS or Lou Gehrig’s disease | Autosomal dominant mutation in superoxide demutase (SOD1) | Death of motor neurons of the motor cortex, brain stem and spinal cord | Fibroblasts |
Friedreich’s ataxia (FRDA) | GAA trinucleotide repeat in the first exon of the frataxin gene gets expanded | Accumulation of mitochondrial iron, specific enzymes in mitochondria become defective, sensitivity to oxidative stress increases, cell death mediated by free radicals | Fibroblasts |
Lesch-Nhyan syndrome (carrier state) | Deficiency of hypoxanthine guanine phospho ribosyl transferase (HPRT) | Over-production of uric acid, low or medium level of mental retardation, megaloblastic anemia is frequent | Dermal fibroblasts |
Shwachman-Bodian-Diamond syndrome (SBDS) | Mutations in the Shwachman-Bodian-Diamond syndrome (SBDS) gene | Exocrine pancreatic insufficiency, predisposition to leukemia, hematopoietic dysfunction | Fibroblasts |
Gaucher’s type III | Deficiency of acid hydrolase, β-glucocerebrosidase, or glucosylceramidase | Myoclonal epilepsy, nerve deafness | Fibroblasts |
Generation of human prostate and urinary tract cells | NA | NA | Human prostate and urinary tract cells |
Adenosine deaminase deficiency-related severe combined immunodeficiency (ADA-SCID) | Defects in Adenosine deaminase (AD) gene | Impaired development and functioning of T, B, and NK cells; complete absence of humoral and cellular immunity; recurrence of infections. | Bone Marrow derived mesenchymal cells |
Type 1 diabetes mellitus (DM) | Progressive β-cell destruction | Long term micro and macro-vascular complications. | Fibroblasts |
Hemophilia A | Deficiency of factor VIII | Decreased protein production, inefficient clotting of blood | Fibroblasts |
Familial hypercholestrolaemia | Mutation in low density lipoprotein receptor (LDLR) gene | Deficiency of LDL-receptor mediated uptake of cholesterol | Dermal fibroblasts |
Spinal muscular atrophy | Mutation in survival of motor neuron 1 (SMN1) gene | Paralysis, muscle weakness and often death | Fibroblasts |
Hutchinson-Gilford progeria syndrome | Point mutations in lamin A | Premature atherosclerosis, vascular smooth muscles gets degraded | Fibroblasts |
Hutchinson-Gilford progeria syndrome | Point mutations in lamin A | Premature atherosclerosis, vascular smooth muscles gets degraded | Fibroblasts |
Drug Discovery
These are the basic reasons for using iPSCs for drug discovery.
- iPSCs have led to the advancement of numerous high throughput and combinatorial screening technologies thus supplementing the role of stem cell models in drug discovery.
- iPSCs based methodology is complementarity to drug discovery or prediction of toxicity via animal models. Animals or in-vitro animal derived cells are used as testing systems but are limited by their inability to replicate the “exact” human physiological conditions and related phenotypic attributions.
- Animal models are not good testing models for drug toxicity as a chemical may be toxic to an animal but may not be toxic to a different animal. For carcinogenic agents as well, different agents pose different levels of carcinogenicity in different animals, for example, formaldehyde is more carcinogenic to rats as compared to mice
- A newly discovered drug or therapy must be tested on human cells or human test models itself. These reasons make it more important to be able to use the systems closer to humans. Moreover, these studies need to be done in a system where the results could be directly extrapolated to humans. The use of iPSCs helps to extrapolate and mimic
- Finally, iPSC technology offers the prospect of capturing cells derived from a large number of specific types of pre-diagnosed adult patients, potentially at any age, and a correspondingly large number of controls in a format that can support an industrial level of screening, efficacy, and safety studies.
Patient-derived somatic cells (for example, fibroblasts) can be reprogrammed to generate iPSCs carrying a disease-specific genetic aberration. These cells can then be differentiated into the disease-affected cell type (for example, neurons in neurodegenerative diseases). After the establishment of a cellular disorder model with disease-specific phenotypes, three main strategies are commonly used: high-throughput screening (HTS) of drugs, the candidate drug approach or patient-specific therapy. In HTS, a very large number of compounds are tested on the differentiated cells, followed by phenotype re-evaluation. This method is extremely valuable for identifying novel therapies in vitro, by using large libraries of compounds. By contrast, both the candidate drug approach and the patient-specific therapy use a small number of potential drugs to attenuate the disease. These approaches are useful when the disease mechanism is known and potential therapies are available. Drugs found by both the HTS and candidate drug approaches usually require substantial safety assays before being prescribed to patients, whereas drugs already approved by regulatory agencies can be immediately prescribed for treatment.
Use of iPSC-Based Assays in Testing Drugs for Toxicity
Before using drugs on humans, their toxic effects must be properly evaluated for a safe administration of those drugs which is very costly. Only 10% of the drugs that enter clinical trials are able to reach market approval stage. The cost of developing a drug is increasing with the estimated cost of whole process being US $1.2–1.7 billion per drug compound. The development of 30% of the medicines was abandoned because of lack of efficacy and 30% due to concerns associated with safety (cardiotoxicity, hepatotoxicity).
iPSC-derived cells of various tissue types can be cultured in large grids and assayed for toxicity in a manner analogous to high-throughput screens for drug discovery. Using this approach, iPSC technology can be integrated into the current paradigm for drug development as part of safety testing in the early phases of clinical trials.
The benefits of using iPSCs in toxicity testing are:
- This method offers an additional line of safety by testing in nontarget tissues, informing clinical trials before harm to human subjects or patients.
- In addition to the safety of testing therapeutics on iPSC-derived cells, there is also the benefit of cost savings. In recent years, the estimated cost of developing and testing a new drug can potentially introduce savings in the costs of development, possibly generate more candidate compounds in a shorter amount of time, and test for life-threatening toxicity in a multitude of tissues. This body of knowledge may help prevent the costly recall of already-approved drugs and develop a new generation of safer drugs using an alternate, less expensive strategy.
Use in Neurotoxicity
Human neurons derived from iPSCs can be attractive models to study the neurotoxicity. The iPSC-derived neurons exhibit functionality and behavior of mature neurons and are available in large quantities. The neurotoxicity test models will allow for studying on one hand the adverse effect of drug candidates on neuronal cells and on the other hand the general neurotoxicity in assays that are well suited for screening of lead compounds and potentially important for reducing animal experimentation and the cost of preclinical development.
Currently, cell lines such as PC12 are typically used for the analysis of calcium signaling with the purpose of determining the complex cellular changes triggered by environmental and pharmacologic neurotoxicants.
Use in Cardiotoxicity
Cardiotoxicity can lead to the formation of reactive oxygen species (ROS), apoptosis, altered contractibility, change in cardiac rhythm, and altered cardiac gene expression, which can be life threatening or may lead to long-term alterations of cardiovascular functions. Of the 40% of drug failures during the clinical trials[i],19% drug withdrawal has been observed due to cardiotoxicities.
In many cardiotoxicity cases, a direct interaction of drugs with specific ion channels expressed by the cardiomyocytes leads to alteration in ion conduction through these specific channels. Drug effects on potassium currents could lead to QT-prolongation, potentially fatal arrhythmias and sometimes cardio-muscular damage without affecting ion channels.
The ESC- and iPSC-derived cardiomyocytes are considered to be well suited to study the effects of compounds which do not interfere with the ion channel functions but still cause cardiotoxicity, an effect that cannot be revealed by using the conventional cell line and receptor overexpression-based approaches[ii].
- Kola I, Landis J. Can the pharmaceutical industry reduce attrition rates? Nature Reviews Drug Discovery. 2004;3(8):711–715.
- Braam SR, Tertoolen L, van de Stolpe A, Meyer T, Passier R, Mummery CL. Prediction of drug-induced cardiotoxicity using human embryonic stem cell-derived cardiomyocytes. Stem Cell Research. 2010;4(2):107–116.
Copyright: No reproduction or republication without written permission.